Introduction
Aspartate transcarbamoylase, commonly referred to as ATCase, is a pivotal enzyme in the biosynthesis of nucleoside triphosphates, specifically cytidine triphosphate (CTP). This enzyme provides the first step in the metabolic pathway leading to the formation of CTP from its precursors. One of the remarkable features of ATCase is its regulation through a feedback mechanism by CTP itself, acting as an allosteric regulator. In this article, we will delve deeply into the structure and function of ATCase, how its quaternary structure is organized, and how substrate binding affects its activity.
Overview of Aspartate Transcarbamoylase (ATCase)
What is ATCase?
Aspartate transcarbamoylase is an allosteric enzyme that plays a crucial role in nucleotide metabolism, specifically catalyzing the reaction between aspartate and carbamoyl phosphate to form carbamoyl aspartate and orthophosphate. This step is integral for the synthesis of pyrimidine nucleotides, which are essential for DNA and RNA synthesis.
Allosteric Regulation
ATCase is an example of an allosteric enzyme, where its activity can be modulated by the binding of regulatory molecules. CTP has been found to bind to specific allosteric sites on the enzyme, reducing its catalytic activity. This feedback inhibition is crucial in maintaining the balance of nucleotide levels within the cell.
Structural Organization of ATCase
Quaternary Structure
ATCase exhibits a quaternary structure, composed of multiple subunits. The enzyme consists of two main types of structures:
- Catalytic Trimers: These are groups of three identical catalytic chains that contain the enzyme's active sites.
- Regulatory Dimers: These are pairs of identical regulatory chains where CTP binds to modulate the enzyme’s activity.
As a result, the overall structure of ATCase comprises two catalytic trimers and three regulatory dimers, totaling twelve individual subunits that work in concert.
The 3D Structure
When visualizing ATCase, the regulatory dimers appear in red, while the catalytic trimers are shown in orange. The arrangement provides a triangular structure, with the regulatory dimers situated between the catalytic trimers.
Interaction Between Catalytic and Regulatory Subunits
Role of Zinc Atoms
Each regulatory subunit features a zinc atom at its interface where it interacts with the catalytic subunits. This metal atom is vital for stabilizing the interaction between these two subunits, thus enhancing the enzyme's functionality.
When a regulatory dimer binds CTP, it alters the configuration of the catalytic trimers, impacting the enzyme’s activity.
Mechanism of Substrate Binding
Active Site Identification
Research on ATCase has revealed the location and dynamics of its active sites. Scientists employed a substrate analog known as PALA (N-phosphonacetyl-L-aspartate) to mimic the substrate’s binding. This irreversible inhibitor resembles an intermediate in the enzymatic process, allowing for the exploration of conformational changes within the enzyme upon binding.
Structure-Function Relationship
Upon binding of the PALA molecule, structural changes occur, highlighting the interface between catalytic chains. The presence of these bound inhibitors reveals how active sites are located at the boundaries between pairs of catalytic subunits. Given that ATCase features two trimers, it possesses six active sites, underscoring its ability to catalyze multiple reactions simultaneously.
Conformational States and Cooperativity
T-State vs. R-State
Just like hemoglobin, ATCase can exist in two primary structural states - the tense (T-state) and relaxed (R-state). In the absence of substrate binding, the enzyme remains in the T-state, characterized by a low affinity for substrates and reduced catalytic activity. However, as substrate analogs like PALA are added, the enzyme transitions to the R-state, where the arrangement becomes more relaxed, and the affinity for substrates increases dramatically.
Effect of Allosteric Regulation
The R-state allows for enhanced catalytic efficiency due to a higher affinity for substrates, a phenomenon known as cooperativity. Thus, as more active sites become occupied, the quaternary structure undergoes significant conformational changes that further promote substrate binding, creating a positive feedback loop that enhances enzymatic activity.
Conclusion
In summary, Aspartate Transcarbamoylase (ATCase) is a crucial allosteric enzyme that plays a key role in nucleotide biosynthesis. Its unique quaternary structure, regulated by CTP and characterized by cooperative binding, allows for efficient catalysis of important biochemical reactions. Understanding the structure and function of ATCase not only provides insight into metabolic pathways but also highlights the complex mechanisms of enzymatic regulation. Future research will further elucidate the details of allosteric regulation and its implications in cellular metabolism.
aspartate transcarbamoylase or ATC ace is this enzyme found sign era cells that catalyzes the first step in the
biosynthesis of per imaging based nucleoside triphosphate molecules such as CT p or cytidine triphosphate and
this enzyme is actually controlled by these CTP molecules because they can ultimately go back and bind on to
special regulatory sites allosteric sites found on the enzyme and that inhibits the activity of the enzyme and
so that's why we call aspartate transcarbamoylase and allosteric enzyme now what we want to focus on in this
lecture is the actual structure of aspartate transcarbamoylase and then we once discuss what happens to that
structure of the enzyme once the substrate molecule actually binds onto the active side of the enzyme so let's
begin by describing the structure now this enzyme has quaternary structure and what that means is it consists of
multiple subunits and there are two types of multi subunit structures one of them we call a catalytic trimer and we
call it catalytic because it contains the active sites the other one is called a regulatory dimer and we call this one
regulatory because that's where that regulatory molecule binds to the CTP molecule binds to the regulatory dimer
as we'll see in the next lecture so each one of these catalytic trimers actually consists of three individual but
identical catalytic chains and that's why we call it a trimer so C 3 where C stands for catalytic and 3 stands for
trimer and we actually have two of these catalytic trimers so we have a total of 2 x 3 so six of these individual about
identical catalytic chains on the other hand we have three of these regulatory diamonds and each dimer consists of two
identical chains two identical regulatory change and so we also see that we have three times two so six of
these regulatory chains so we have six catalytic chains we have six regulatory chains to make a total of 12 of these
subunits that make up the coronary structure of aspartate transcarbamoylase and if we examine the three-dimensional
structure of this molecule from top to bottom this is basically what we see so these red dimers are the regulatory
dimer so each one of these diamonds consists of want to want to wants you of these regulatory chains and this entire
orange structure constitutes a single catalytic trimer so we have 1 2 3 of these orange subunits each one of these
orange subunits is a catalytic chain and three of these catalytic chains arranged in this triangular format make up that
catalytic trimer now because we're examining the structure from top to bottom the other trimer is hiding
beneath this trimer so if we take this structure and flip it this way we're going to see this on top and the other
trimer is basically found on the bottom so we have a total of two Catalan trainers and three of these diamonds on
the sides so we have this triangular form as shown in this diagram now the question is what exactly is the
interaction like between regulatory dimers and these catalytic trimers well it turns out that each one of the
regulatory chains in each one of these dimers interacts with one of these catalytic chains in each one of these
catalytic trimers and this interaction is made better it is amplified by the presence of a metal atom of a zinc metal
atom so if we examine so let's take a look at one of these red structures let's say we're examining this
regulatory structure here so this is basically what we're going to see so we have a bunch of these beta chains we
have some of these alpha chains and right about here at the interface between the red regulatory
chain and this orange catalytic chain we're going to find a zinc atom and that zinc atom interacts with the cysteine
residues with four cysteine residues and that basically amplifies it makes better the interaction between the orange chain
the catalytic chain and the red chain that regulatory change so each our regulatory subunit contains a zinc
domain that contains the zinc metal atom that interacts with the C subunit the catalytic subunits so again each one of
these red regulatory chains in the dimer interacts with one of these catalytic chains in that catalytic trimer via this
metal atom that is present within the end portion the interface section of each one of these regulatory chains now
when scientists were basically studying this molecule how exactly that they actually discover where the active site
is found within this molecule well instead of actually using the substrate molecules for this enzyme they used an
inhibitor a irreversible inhibitor to this enzyme they synthesized an irreversible inhibitor that resembles
the two substrate molecules of this particular enzyme so remember what a TCAs
actually does it catalyzes the conversion of a molecule called aspartate and a second molecule called
carbamoyl phosphate so it combines these two molecules to form the and carbamoyl aspartate as well as a single ortho
phosphate molecule now what this molecule does the science is created so scientists created this irreversible
inhibitor a by substrate analogue known as the pala molecule which stands for an phospho acid salal aspartate and the
structure of this Polamalu resembles the intermediate molecule that is formed in the by synthesis of the CTP
molecule and this is what pala actually looks like so Paolo resembles an intermediate in the reaction pathway and
is therefore a highly potent a highly effective inhibitor it binds onto the active side because it resembles the
bi-substrate analog that is formed as an intermediate in this particular reaction and so once it binds on to the active
side it binds irreversibly and it does not let go and that inhibits the activity of this enzyme now once we
actually bind the pala molecule to the active side that also that not only tells us well that where the active site
is down but it can also be used to basically study what kind of structural changes actually take place within the
enzyme as we'll see in just a moment now one thing I'd like to point out about the active side of the enzyme wherever
that active site is we have these three negative charges on this pala molecule and the fact that we have three negative
charges basically implies that inside the active site of this enzyme will find residues that contain positive charges
so basically those positive charges in the residue interact with the negative charges of this bi-substrate analog
molecule the pala molecules so the next question is what exactly did scientists see when they mix the pala with this
enzyme well what they saw was where that pala actually bound to was at the interface between any pair of these
catalytic chains within that trimer so the Pauline hibbott ER binds on to a region located on the boundaries of each
pair of catalytic subunits in any one of those catalytic trimers and because we have three of these boundaries between
the pairs in any given trimer we see that we three of these chain are three of these
active sites in a trimer and because we have two trimers that implies we must have six active sites so we see that the
quaternary structure of the eighty CAS actually consists of six individual active sites and that means if all those
active sites are occupied at some given moment in time six of these reactions are taking place at the same exact
moment in time so this is where Paulo bus so this is let's suppose the pala molecule it binds
unto this location the first active side this location the second active side and the third active site as well and of
course if we flip it this way we're going to see the other catalytic trimer and it also contains six
it also contains three of these active sites now the final question I'd like to explore is what did scientists actually
see when that pala molecule was bound on to the active site what types of structural changes actually took place
in the quaternary structure of this ATC ace allosteric enzyme well just like as we discussed in hemoglobin when the
oxygen molecules are not balanced of the heme groups of hemoglobin that hemoglobin molecule eggs exists in the
t-state in the tense day and what that means is the entire quaternary structure is
constrained and so the affinity of the heme groups for oxygen is very low and in the same analogous way because this
allosteric enzyme basically exhibits cooperativity it also exists in the t-state
as well as in the r-state so when the pala molecule he is absent from the environment that means none of the
active sites are actually occupied and so the entire quaternary structure of this enzyme will exist in the t-state it
will be very tense and very constrained so that means the affinity of all the active sites for the substrate will be
low and so it will display a low catalytic activity but as those substrate molecules and in this
particular case as the by substrate analog the pala molecule begins to bind onto the active sides of the enzyme what
begins to happen is the trimers basically begin to move away from one another and as more and more active
sites are filled the trimers move even farther and as they move they rotate and they cause the dimers to also rotate and
move and that creates a quaternary change in the coronary structure of that molecule and that causes it to basically
go from the t's state to the relaxed state it basically becomes less constrained more relaxed and the
affinity of the active sites for the substrate molecule increases as this actually takes place so once again as
the substrate binds in this case as the pollen hibbett are binds unto the active site it causes the two trimers to move
farther apart and rotate which in turn causes the regulatory subunits to also move and therefore the entire structure
seems to expand upon the binding of that substrate molecule to the active site and so as the active sites become filled
we see that the tense stay the t's state transitions into the RF state and the equilibrium basically shifts so to see
what we mean let's take a look at the following diagram so we have this equilibrium that exists between the t
state and the Arth state now before we add any pala molecules when they're not present in the
environment in the absence of the pala substrate or the actual substrate aspartate or the carbamoyl phosphate the
quaternary structure exists in the t's state and in this stage the enzyme has a low affinity for the substrate molecules
and will display a low cattle activity on the other hand as we begin to add the pala molecules they begin to
slowly add into the active sites found in those catalytic trimers and as they begin to fill those active sites what
that does is it moves so this is one primer this is a second trimer as the pala begins to bind onto the trimer
active sites the entire trimers begin to move apart as they move apart they rotate and they cause the movement of
these regulatory sites and so the entire molecule basically expands it becomes more relaxed so it becomes less
constrained and what that means is the active sites of the other the other active sites which are unoccupied will
basically increase their affinity for the substrate molecule and they will be much more likely to actually bind onto
those substrate molecules and so ultimately as more of the active sites become filled with those substrate
molecules the equilibrium basically shifts to the r-state and what that means is at a high concentration of
substrate when most of the active sites will be filled by the substrate molecule all the enzymes will exist predominantly
in the r-state but in the absence of the substrate molecule when none of the active sites are filled by the substrate
molecules that means all the enzymes will predominate in the t-state now the thing about the RS state is the
our state describes this relaxed state relaxed structure where there is not so much constraint and so the affinity of
the active site for the substrate is high and that represents a high catalytic activity a high catalytic rate
now what we still haven't discussed is where the CTP actually binds to and how exactly does
the binding of the CTP molecule to the regulatory dimer that's where it binds to how exactly is the binding
the CTP on say these regulatory dimers actually inhibits affects the activity of that enzyme this is what we actually
want to focus on in the next lecture and we're also going to discuss what this molecule or how this molecule actually
exhibits the process of cooperativity so remember the reason this molecule exhibits cooperativity is because it
consists of these twelve different sub
Heads up!
This summary and transcript were automatically generated using AI with the Free YouTube Transcript Summary Tool by LunaNotes.
Generate a summary for freeRelated Summaries
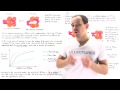
Understanding Aspartate Transcarbamoylase: Cooperativity and Allosteric Regulation
Dive into the functions of aspartate transcarbamoylase, its cooperativity, and the roles of CTP and ATP in regulation.
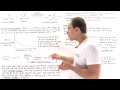
Understanding Allosteric Regulation: The Role of Aspartate Transcarbamoylase
Explore how aspartate transcarbamoylase is regulated and its importance in synthesizing nucleotides.
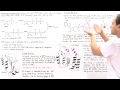
Understanding Nucleoside Monophosphate Kinases: The Role of Enzymes in Energy Transfer
Explore the role of nucleoside monophosphate kinases in energy transfer and enzyme catalysis, including mechanisms like metal ion catalysis.
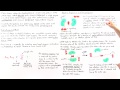
Understanding Protein Kinases: Functionality, Specificity, and the Role of Protein Kinase A
Explore protein kinases, their functions, specificity, and the critical role of Protein Kinase A in cellular processes.
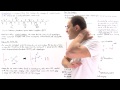
Understanding the Catalytic Properties of Enzymes: The Case of Chymotrypsin
Explore the catalytic mechanisms of enzymes with a focus on chymotrypsin's role in peptide bond hydrolysis.
Most Viewed Summaries
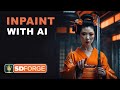
Mastering Inpainting with Stable Diffusion: Fix Mistakes and Enhance Your Images
Learn to fix mistakes and enhance images with Stable Diffusion's inpainting features effectively.
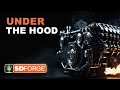
A Comprehensive Guide to Using Stable Diffusion Forge UI
Explore the Stable Diffusion Forge UI, customizable settings, models, and more to enhance your image generation experience.
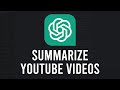
How to Use ChatGPT to Summarize YouTube Videos Efficiently
Learn how to summarize YouTube videos with ChatGPT in just a few simple steps.
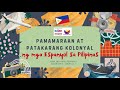
Pamaraan at Patakarang Kolonyal ng mga Espanyol sa Pilipinas
Tuklasin ang mga pamamaraan at patakarang kolonyal ng mga Espanyol sa Pilipinas at ang mga epekto nito sa mga Pilipino.
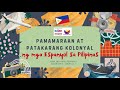
Pamamaraan at Patakarang Kolonyal ng mga Espanyol sa Pilipinas
Tuklasin ang mga pamamaraan at patakaran ng mga Espanyol sa Pilipinas, at ang epekto nito sa mga Pilipino.