Introduction
Thermodynamics is a fascinating field of study that delves into the physical laws governing energy and its transformations. A key player in thermodynamics is the PV diagram, a graphical representation of the relationship between pressure (P) and volume (V) of a system. In this article, we will dissect the intricate dynamics of the PV diagram, the significance of enthalpy, and how these concepts blend to define the heat content during chemical reactions. From understanding work done by the system to exploring state variables, we aim to establish clarity on these critical thermodynamic principles.
What is a PV Diagram?
The PV diagram is a crucial tool in thermodynamics that allows us to visualize the relationship between pressure and volume as a system undergoes changes. In a typical PV diagram:
- The x-axis represents volume (V)
- The y-axis represents pressure (P)
Quasistatic Processes
When considering transitions between states in a PV diagram, it’s essential to note that if we move from one point to another quasistatically—meaning we maintain the system near equilibrium—our state variables will always be defined. This quasistatic process forms a path on the graph, where:
- The work done by the system is represented as the area under the curve of this path.
Work Done in Various Paths
Moving from one state A to state B on the PV diagram, we can observe different paths:
- A return path to the original state results in the work done by the system being captured within the area enclosed by the path.
- The net work done, therefore, becomes crucial as it can be depicted as the area inside this defined path on the PV graph.
Understanding Internal Energy
Equally important is understanding internal energy (U)—a state function defined by the first law of thermodynamics. According to this law, the change in internal energy ( (\Delta U)) can be expressed as:
- (\Delta U = Q - W) Where:
- (Q) is the heat added to the system
- (W) is the work done by the system
If the system returns to its original state in the PV diagram, both the pressure and volume revert to their initial values, resulting in zero change in internal energy ( (\Delta U = 0)). This indicates that the heat added effectively balances the work done within the cycle.
The Concept of Enthalpy
Definition of Enthalpy
Moving forward, we encounter the concept of enthalpy (H), a state variable defined as:
- (H = U + PV) The inclusion of the pressure-volume product in this definition allows us to grasp how enthalpy serves as a more useful measure of heat content under specific circumstances.
Changes in Enthalpy
The change in enthalpy ( (\Delta H)) can be expressed as:
- (\Delta H = \Delta U + P \Delta V + V \Delta P) This formulation reveals that the change in enthalpy reflects not only changes in internal energy but also the work associated with pressure and volume changes.
Enthalpy and Heat at Constant Pressure
Constant Pressure Conditions
One key takeaway from our exploration into enthalpy is its applicability in systems at constant pressure. When pressure remains constant, the work done by the system does not vary throughout the process, providing a balance between the heat added and the change in enthalpy. Therefore, under these conditions:
- (\Delta H = Q) or clarified, heat added equals change in enthalpy.
Practical Implications in Chemistry
The significance of this constant-stage context arises particularly in chemical reactions occurring in open systems, where reactions typically occur at atmospheric pressure. Here, understanding enthalpy becomes vital as:
- It provides a clear insight into whether reactions require heat to proceed or release heat during the process.
Visualizing Constant Pressure on a PV Diagram
In a PV diagram where pressure is held constant, changes happen along a horizontal line:
- The area under the curve shrinks since there’s no effective work being done by changing the pressure.
- Thus, examining such a scenario visually reinforces the understanding that under constant pressure, enthalpy equates effectively to heat added.
Why is Enthalpy Important?
Relevance to Chemical Reactions
Thus, we can conclude that understanding enthalpy allows chemists to predict reaction behaviors:
- If the enthalpy increases during a reaction, it indicates an endothermic reaction (heat absorption).
- Conversely, if the enthalpy decreases, it indicates an exothermic reaction (heat release).
Applications in Real-Life Scenarios
- In laboratory environments, knowing the enthalpy changes allows researchers to design experiments more efficiently, considering necessary conditions for reactions.
- Moreover, applications extend beyond chemistry, influencing various fields like engineering, environmental science, and materials science.
Conclusion
In conclusion, the PV diagram is not merely a representation of pressure and volume but a robust tool that aids in understanding the various transformations in thermodynamic systems. The introduction of enthalpy as a state variable extends our capability to analyze heat transfer during processes under constant pressure. Understanding these principles is pivotal not just for theoretical excellence in thermodynamics but also for practical implications in the real world, particularly in chemical reactions. As such, the relationship between pressure, volume, heat, and enthalpy stands as an essential focal point in mastering thermodynamic principles.
Let me draw a good old PV diagram. That's my pressure axis, this is my volume axis. Just like that, I have pressure and volume.
I showed several videos ago that if we start at some state here in the PV diagram, right there, and that I change the pressure and the volume to get to another state, and I do it
in a quasistatic way, so essentially I'm always close to equilibrium, so my state variables are always defined, I could have some path that takes me to some other state
right there. And this is my path. I'm going from this state to that state.
And we showed that if I just did this, the work done by the system is the area under this curve. And then if I were to move back to the previous state,
and then if I were, you know, by some path, just some random path that I happened to be drawing, the work done to the system would be the area under this light blue curve.
So the net work done by the system ended up being the area inside of this path. So this is-- let me do it in different color.
The net work done would be the area inside of this path when I go in this clockwise type of direction. So this is the net work done by the system.
And now, we also know that if we're at some point on this PV diagram, that our state is the same as it was before. So if we go all the way here, and then go all the way back,
all of our state variables will not have changed. Our pressure is the same as it was before. Our volume was the same as it was before because we went all
the way back to that same point on the PV diagram. And our internal energy is also the same point as it was before, so our change in internal energy over this
path, you're going to have a different internal energy here than you had here, but when you go around the circle and you get back, your change in internal
energy is equal to zero. And we know that our change in internal energy is defined as, and this is from the first law of thermodynamics, the heat
added to the system minus the work done by the system. Now, if we go on a closed loop on our PV diagram, then what's our change in internal energy?
It's zero. So we get zero change in internal energy, because we're at the same state is equal to the heat applied to the system
minus the work done or-- and I've done this little exercise multiple times. I think is probably the fourth or fifth time I'm doing it.
We get that the heat added to the system, if we just add w to both sides, is equal to the work done by the system. So this area inside of this path, I already said, it's the
work done by the system, and if you don't remember even where that came from, it was, remember, pressure times volume times change in volume is a little incremental change
in work, and that's why it relates to the area. But we've done that multiple times. I won't go there just yet.
So if you have any area here, some heat was added to the system, some net heat, right? Some heat was added here, and some heat was probably taken
out here, but you have some net heat that's added to the system. And I use that argument to say why heat isn't
a good state variable. Because-- and I had a whole video on this-- that if I define some state variable, let's just say, heat content.
Let's say I want to define some state variable heat content. And I would say that the change in heat content would,
of course, be equal to the change in heat. That's what I'm defining. If I'm adding heat to the system, my heat
content should go up. But the problem with that heat content state variable was that, let's say over here, I say that the heat content is
equal to 5. Now, I just showed you that if we go on some path here and we come back, and there's some area in this little path that
I took, that some heat was added. So let's say that this area right here, so this is q is equal to the work done by the system, let's say
it's equal to 2. So every time, if I start at heat content is equal to 5, that's just an arbitrary number, and I were to do this
entire path, when I go back, the heat content would have to be 7. And then when I go back and do it again, my heat content
would have to be 9. And it would have to increment by 2 every time I do this exact path.
It would have to increment by the amount of area that this path goes around. So heat content can't be a state variable, because it's
dependent on how you got there. A state variable-- and remember this. In order to be a state variable, if you're at this
point, you have to have the same value. If your internal energy was 10 here, when you do the path and you come back, your internal energy will be 10 again.
That's why internal energy is a valid state variable. It's dependent only on your state. If your entropy was 50 here, when you soon. go back and you
do all sorts of crazy things, and you come back to this point, your entropy is once again 50. If your pressure here is 5 atmospheres, when you come
back here, your pressure will be 5 atmospheres. Your state variable cannot change based on what path you took.
If you're at a certain state, that's all that matters to the state variable. Now this heat content didn't work, and that's why we
actually led into some videos where I divided by t and we got entropy, which was an interesting variation. But that's still not satisfying.
What if we really wanted to develop something that could in some way be a state variable, but at the same time measure heat?
So obviously, we're going to have to make some compromises, because if we just do a very arbitrary kind of heat content variable, then every time you go around this,
it's going to change. That's not a valid state variable. So let's see if we can make up one.
So let's just make up a definition. Let's call my new thing that I'm going to try to maybe approximate heat, let's call it h, and just as a little bit
of a preview, we're going to call it enthalpy. And let's just define it. I'm just playing around.
Let's just define it as the internal energy plus my pressure times my volume. So then what would my change in enthalpy be?
So my change in enthalpy will be, of course, the change of these things. But I could just say, that's my change in my internal
energy plus my change in pressure times volume. Now, this is interesting. And I want to make a point here.
This, by definition, is a valid state variable. Why is it? Because it's the addition of other state variables, right?
At any point in my PV diagram, and it's also true if I did diagrams that were entropy in temperature or anything that dealt with state variables, at any point on my diagram, u is
going to be the same, no matter how I got there. p is by definition going to be the same. That's why it's at that point.
v is definitely going to be the same point. So if I just add them up, this is a valid state variable because it's just the sum of a bunch of
other valid state variables. So let's see if we can somehow relate this thing that we've already established as a valid state variable.
From the get-go, from our definition, this works because it's just the sum of completely valid state variables.
So let's see if we can relate this somehow to heat. So we know what delta u is. If we're dealing with all of the internal energy or the
change in internal energy, and I'm not going to deal with all the other chemical potentials and all of that, it's equal to the heat applied to the system minus the work done by the
system, right? Let me put everything else there. The change in enthalpy is equal to the heat applied
minus the work done-- that's just the change in internal energy-- plus delta PV. This is just from the definition of my enthalpy.
Now this is starting to look interesting. What's the work done by a system? So I could write change in h, or enthalpy, is equal to the
heat applied to the system minus-- what's the work done by a system? If I have some system here, it's got some piston on it,
you know, if we're doing it in a quasistatic, I have those classic pebbles that I've talked about in multiple videos.
When I apply heat or let's say I remove some of these pebbles, so I'm at a different equilibrium, but what's actually happening?
When is the work being done? You have some pressure being applied up here, and this piston is going to be moving up, and your volume is going
to increase. And we showed multiple videos ago that the work done by the system can be, and you can kind of view this as the
volume expansion work, it's equal to pressure times change in volume. And let's add the other part.
So this was our change in internal energy. And I had several videos where I show this. And now let me add the other part of the equation.
So our enthalpy, our change in enthalpy, can be defined by this. Now.
Something interesting is going on. I said that I wanted to define something, because I wanted to somehow measure heat content.
My change in enthalpy will be equal to the heat added to the system, if these last two terms cancel out. If I can somehow get these last two terms to cancel out,
then my change in enthalpy will be equal to this, if somehow these are equal to each other. So under what conditions are these equal to each other?
Or another way, under what conditions is delta pressure times volume equal to pressure times delta volume? When does this happen?
When can I make this statement? Because if I can make this statement, then these two terms are equivalent right here, and then you my change
in enthalpy will be equal to the heat added. Well, the only way I can make this statement is if pressure is constant.
Now why is that? Let's just think about it mathematically. If this is a constant, then if I just change-- you know, if
this is just 5, 5 times a change in something is the same thing as the change in 5 times that thing, so it just mathematically works out.
Or if you view it another way, if this is a constant, you can just factor it out, right? Well, if I said, the change in 5x, that would be equal to 5
times x final minus 5 times x initial. And you could say, well, that's just equal to 5 times x final minus x initial.
Well, that's just equal to 5 times the change in x. It's kind of almost too obvious for me to explain. I think sometimes when you overexplain things, it might
become more confusing. So this applies-- and the 5 I'm just doing as the analogy for a constant.
So if pressure is constant, then this equation is true. So if pressure is constant-- so this is a key assumption-- then if heat is being applied in a constant pressure
system-- so we could write it this way. I'll write it multiple times, because this is key. If pressure is constant, then our definition, our little
thing we made up, this enthalpy thing, which we defined as internal energy plus pressure and volume, then in a constant pressure system, our change in enthalpy we just
showed is equal to the heat added to the system because all of these two things become equivalent under constant pressure, so I should write that.
This is only true when heat is added in a constant pressure system. So how does this gel with what we did up
here on our PV diagram? What's happening in a constant pressure system? Let me draw our PV diagram.
That's P, that's V. So what's happening in constant pressure? We're at some pressure right there.
So if we're under constant pressure, that means we can only move along this line. So we could go from here to there and back to there, or we
could go from there to there, back to there. So we could go there, all the way there, and then go back. But what do we see about this?
Is there any area in this curve? I mean, there is no curve to speak of, because we're staying in a constant pressure.
We've kind of squeezed out this diagram. We've made the forward path and the return path the same exact path.
So because of this, you don't have that state problem because no net heat is being added to the system when you go from this point all the way to this point and then back to
this point. So because of that, you can kind of see visually that enthalpy in a constant pressure, when you're not
moving up and down in pressure, is the same thing as heat added. So you might say, hey Sal, this was a bit of a
compromise, constant pressure, you know, that's a big assumption to make. Why is this useful at all?
Well, it's useful, because most chemical reactions, especially ones that occur in an open beaker, or that might occur at sea level, and that should be a big clue, they
occur at constant pressure. You know, if I'm sitting at the beach, and I have my chemistry set, and I have some beaker of something, and I'm
throwing other stuff into it, and I'm looking for a reaction or something, it's a constant pressure system. This is going to be atmospheric pressure.
1 atmosphere. I'm sitting at sea level. So this is actually a very useful concept for everyday
chemical expressions. It might not be so useful for engines, because engines always have pressure changing, but it's very useful for
actual chemistry, for actually dealing with what's going to happen to a reaction at a constant pressure. So what we're going to see is that this enthalpy, you can
kind of view it as the heat content when pressure is constant. In fact, it is the heat content
when pressure is constant. So somehow-- well, not somehow, I showed you how-- we were able to make this definition, which by
definition was a state variable, because it was the sum of other state variables, and if we just make that one assumption of constant pressure, it all of a sudden
reduces to the heat content of that system. So we'll talk more in the future of measuring enthalpy, but you just have to say, if pressure is constant, enthalpy
is the same thing as-- and it's really only useful when we're dealing with a constant pressure. But if we have a pressure constant, enthalpy can be
imagined as heat content. And it's very useful for understanding whether chemical reactions need heat to occur or whether they release heat,
so on and so forth. See
Heads up!
This summary and transcript were automatically generated using AI with the Free YouTube Transcript Summary Tool by LunaNotes.
Generate a summary for freeRelated Summaries
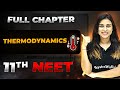
Understanding Thermodynamics: A Comprehensive Overview
This video transcript provides an in-depth exploration of thermodynamics, focusing on key concepts such as enthalpy, entropy, and the laws governing energy transfer. It discusses the significance of spontaneous processes and the relationship between temperature, pressure, and volume in various thermodynamic processes.
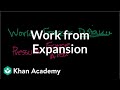
Understanding Internal Energy: Heat and Work in Thermodynamics
Explore how internal energy changes in thermodynamics, focusing on heat transfer and work done in systems.
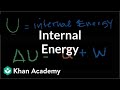
Understanding Internal Energy in Thermodynamics: A Comprehensive Guide
Explore the intricacies of internal energy and its transformations in thermodynamics and learn fundamental principles through practical examples.
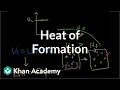
Understanding Enthalpy and Heat of Formation in Chemical Reactions
Explore the concept of enthalpy and its role in chemical reactions, including heat of formation and exothermic vs endothermic processes.
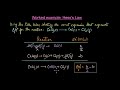
Understanding Hess's Law: A Comprehensive Guide to Enthalpy Changes
Explore Hess's Law and learn how to calculate enthalpy changes with numerical examples. Perfect for chemistry students!
Most Viewed Summaries
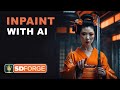
Mastering Inpainting with Stable Diffusion: Fix Mistakes and Enhance Your Images
Learn to fix mistakes and enhance images with Stable Diffusion's inpainting features effectively.
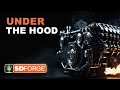
A Comprehensive Guide to Using Stable Diffusion Forge UI
Explore the Stable Diffusion Forge UI, customizable settings, models, and more to enhance your image generation experience.
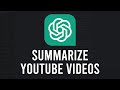
How to Use ChatGPT to Summarize YouTube Videos Efficiently
Learn how to summarize YouTube videos with ChatGPT in just a few simple steps.
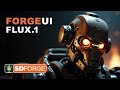
Ultimate Guide to Installing Forge UI and Flowing with Flux Models
Learn how to install Forge UI and explore various Flux models efficiently in this detailed guide.
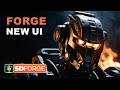
How to Install and Configure Forge: A New Stable Diffusion Web UI
Learn to install and configure the new Forge web UI for Stable Diffusion, with tips on models and settings.