Introduction
Proteases are essential enzymes that play a critical role in various biological processes by catalyzing the hydrolysis of peptide bonds. In our previous discussions, we focused on serine proteases, specifically talking about three major examples: chymotrypsin, trypsin, and elastase. All these serine proteases share a common feature—the catalytic triad composed of serine, histidine, and aspartate residues that facilitate the hydrolysis of peptide bonds. However, proteases exist in several categories beyond just serine proteases, including cysteine proteases, aspartate proteases, and metalloproteases, each with unique mechanisms of action. This article delves into the mechanisms of these different categories of proteases to understand how they differ from, and compare to, serine proteases.
Cysteine Proteases
Mechanism of Action
Cysteine proteases utilize a cysteine residue in their active site as a nucleophilic agent for peptide bond cleavage, unlike serine proteases that use serine. However, cysteine is not inherently a strong nucleophile. For the cysteine residue to function effectively in this manner, it requires the help of another amino acid, commonly histidine, to enhance its nucleophilicity.
Here’s a breakdown of how this transformation occurs:
- Initial State: The cysteine thiol (–SH) group is not a sufficient nucleophile due to its stabilizing hydrogen, which is drawn through a sigma bond.
- Activation by Histidine: When the substrate approaches, the histidine residue pulls the proton (H+) away from cysteine, increasing the electron density on the sulfur atom of cysteine, thereby enhancing its nucleophilicity.
- Nucleophilic Attack: The activated cysteine then attacks the carbonyl carbon of the substrate, leading to the formation of a tetrahedral intermediate, which is stabilized by an oxyanion hole—just like in serine proteases.
Example of Cysteine Proteases
- Caspases: Enzymes that play a crucial role in apoptosis (programmed cell death).
- Cathepsins: Involved in protein degradation and related to immune response.
Aspartate Proteases (Aspartyl Proteases)
Mechanism of Action
In contrast to cysteine and serine proteases, the nucleophilic activity in aspartate proteases arises from water. In aspartate proteases, two residues, aspartate and aspartic acid, collaboratively work to facilitate this process:
- Role of Aspartate: One aspartate acts to activate water, donating its negative charge to create a hydroxide ion, a much stronger nucleophile.
- Electrophilic Activation: The other aspartate residue interacts with the substrate to enhance its electrophilicity.
- Reaction Cycle: Once the water is transformed into a hydroxide ion, it attacks the carbonyl carbon similarly to other proteases, forming a tetrahedral intermediate that also has an oxyanion hole for stabilization.
Example of Aspartate Proteases
- Renin: Regulates blood pressure.
- Pepsin: Operates in the acidic environment of the stomach to digest proteins.
Metalloproteases
Mechanism of Action
Metalloproteases are characterized by the presence of metal ions (usually zinc) in their active site, which are crucial for their function. This is how they operate:
- Metal Ion Activation: The zinc atom interacts with water, creating a more reactive hydroxide ion.
- Base Role: A nearby amino acid, such as glutamate, abstracts the proton from water, facilitating the nucleophilic attack on the substrate’s carbonyl carbon.
- Formation of Tetrahedral Intermediate: The hydroxide ion attacks the carbonyl carbon of the substrate, forming a tetrahedral intermediate, upon which the reaction proceeds to hydrolysis.
Example of Metalloproteases
- Carboxypeptidase A: A digestive enzyme that removes amino acids from protein fragments.
Comparative Overview
All categories of proteases—serine, cysteine, aspartate, and metalloproteases—share a fundamental mechanism of transforming a less effective nucleophile into a strong nucleophile and a poor electrophile into a good electrophile. Despite their differences in the specific chemical residues and the nature of their catalytic mechanisms, their ultimate goal is the efficient hydrolysis of peptide bonds.
- Serine Proteases: Utilize serine as the key nucleophile.
- Cysteine Proteases: Rely on cysteine, activated by histidine.
- Aspartate Proteases: Use water as the nucleophile, activated by aspartate residues.
- Metalloproteases: Employ metalloenzymes to activate water, also creating a strong hydroxide nucleophile.
Conclusion
In conclusion, while serine proteases may serve as the more well-known category of proteolytic enzymes, understanding the mechanisms of cysteine, aspartate, and metalloproteases deepens our appreciation for the biochemical complexity and versatility of proteases overall. By recognizing the common principles that underpin their activity and the specific adaptations that enhance their efficiency, we glean valuable insights into their roles in physiological processes, including digestion, immunity, and cell death regulation.
so far in our discussion on protease is we focused on serine proteases and we discussed three examples of serum
protease so we discussed chymotrypsin trypsin and elastase now we said these three Seor proteases all use the same
catalytic triad so in their active site they have this collection of three amino acids that work together to basically
promote the hydrolysis of peptide bonds and out of these three amino acids it's the serine that acts as the
nucleophilic agent now what about the other categories of protease is so remember we not only have serine
proteases but we also have cysteine protease as a spritzel proteases metalloproteases and we have other
examples of proteases that we're not going to focus on in this lecture so the question is what exactly is the
mechanism of these other proteases and how does the mechanism differ or how does it compare to the mechanism inside
serine proteases so let's begin by briefly discussing cysteine proteases so in cysteine proteases we also have
amino acids in the active side that work together to basically catalyze the cleavage of peptide bonds but unlike
in-ceiling protease is insisting proteases it's the cysteine residue found in the
active side that acts as that nucleophilic agent that will cleave that peptide bond and to see how that
actually works let's take a look at the following diagram so we have a cysteine residue in the active site of some
particular cysteine protease now in the form that we have cysteine here cysteine is not good it is not a strong enough
nucleophile so the sulfur as shown here is not a strong enough nucleophile and it will not be able to attack the carbon
of that carbonyl group on that substrate molecule because this simply isn't a good enough nucleophile so what must
happen is another residue must work together with the same to basically transform it into a better
nucleophile so how does that actually take place well in a similar way to how takes place in serine protease is so we
have a nearby residue for example a histidine and on that histidine we have this nitrogen which contains a lone pair
of electrons on top of that the nitrogen can also have or also has a partial negative charge because it's more
electronegative than the nearby carbon atoms now if we examine this H on the sulfur we see that the H contains a
partial positive charge because the sulfur is more electronegative and the sulfur contains a partial negative
charge so what we see happening is before the substrate actually enters the active side what begins to happen is the
nitrogen because of its higher electron density begins to pull on this h ion and as the H ion is being pulled away on
center nitrogen as shown in this particular diagram these two electrons in the Sigma bond move closer to the
sulphur atom and that increases the electron density the electron cloud around the sulphur and that increases
its ability to act as a nucleophile so as the H atom is being pulled away onto the nitrogen of the nearby histidine
residue we see that these two electrons move closer to the sulfur and the electron density on the sulfur increases
and so as we have this incoming substrate molecule these two electrons attack nucleophilically the carbon on
the carbonyl and that displaces the PI bond and so once the step takes place we form the same type of tetrahedral
intermediate that we formed in the serine protease reaction mechanism and just like in serum protease as we can
have an oxyanion hole that stabilizes the negative charge on that oxygen once we formed a tetrahedral intermediate in
Syst protease is we can also have a similar type of oxyanion hole that stabilizes
that relatively unstable and high-energy tetrahedral intermediate now of course eventually the tetrahedral intermediate
will collapse and after a few processes were going to basically break that peptide bond in an in a similar way to
how we broke the peptide bond in serine proteases now two examples of cysteine proteases are caspases these are those
enzymes involved in the process of programmed cell death so abbott osis as well as cathepsins which are involved in
immunity now let's move on to aspartate protease is also known as a spritzel proteases now the major difference
between cysteine proteases and aspinall proteases is the following insisting proteases as well as the serine
proteases we see that one of the residues inside the active side of the enzyme acts as a nucleophile but in a
special protease the residues don't actually act as nucleophiles instead it's the water that will ultimately act
as the nucleophile now the similarity between let's say cysteine protease and a special protease
is we still have to transform the water into a good nucleophile in the same way that we have to transform the cysteine
into a strong nucleophile before the nucleophilic attack took place in this particular case we see that we're going
to have residues that will transform the water into a better nucleophile now if we examine the active site of a special
proteases we're going to find two residues one of these residues will be aspartic acid and the other one will be
aspartate so this is basically the same thing as this but this is in the deprotonated state and this is in the
protonated state so we have aspartate we have aspartic acid now when water moves into the active side the
negative charge on the aspartate will interact with the positive charge on one of the H atoms so we have a partial
positive charge here because the oxygen contains a partial negative charge and so by the same by the same analogy here
when the nitrogen pulls away the h it gives the electrons in the sulphur and so the electron density on the sulphur
increases and it becomes a better nucleophile here when the negative charge the oxygen pulls away the Aged
it makes the oxygen a better nucleophile because we essentially transform water into a hydroxide and the hydroxide is a
much stronger nucleophile at the same time what happens is the other residue the sponeck acid uses its H to basically
interact with the oxygen of the carbonyl so the oxygen here contains a partial negative charge and the H here contains
a partial positive charge and so as they interact here what begins to take place is we basically make the carbon of the
carbonyl a better electrophile so the ultimate reason why we have these two different we have the aspartate and the
spartax acid is because one transforms the water into a better nucleophile and the other one transforms the incoming
substrate into a better electrophile and now we can have our reaction take place the similar reaction the same type of
reaction that took place here we have this bond breaking forming a bond with carbon attacking the carbon
nucleophilically displacing the PI bond forming that same type of tetrahedral intermediate except now instead of
having a bond between this residue and the carbon we have a bond between the oxygen of the water and this carbon so
inside the active side of these proteases is a pair of aspartic acid residues they work together to transform
water into a good nucleophile so that it can hydrolyze that peptide bond so what happens is
deprotonated aspartic acid that aspartate shown here uses its negative charge to transform the water into a
better nucleophile so we essentially create a hydroxide and then the protonated version of the residue the
sparta castle uses the partially positive hydrogen to basically interact with that partially negative oxygen of
the carbonyl and that creates a good electrophile and now the reaction can take place at a relatively high rate now
two examples of aspartate proteases that we're going to focus on in future lectures is renin and this is basically
the enzyme that is used to control blood pressure as well as pepsin and this is one of the digestive enzymes that is
found in our digestive system and finally let's take a look at metalloproteases so just like the name
implies this has to do with the fact that inside the active site of metalloproteases we actually have unmet
'el atoms and usually the metal atom is zinc so what's the point of the metal atom well for the same exact reason that
we have this residue to basically transform the water into a better nucleophile here we also have a metal
atom to actually bind the water and transform it into a better nucleophile so that the water can basically
hydrolyze that peptide bond so if we examine the active site as shown here let's say we have the zinc metal atom
that is actually down to the active side of that enzyme and so what it does is it interacts with the oxygen as a result of
this being partially negative and this having a positive charge so they form this bond at the same time some type of
nearby residue acts as a base and usually the residue is glutamate so this acts as a base and basically grabs off
the Aged it pulls the H away from this oxygen and that transforms the oxygen into a much better nucleophile so it
creates that hydroxide and this can act as a nucleophile attacking the carbon of the carbonyl and once
again forming a tetrahedral intermediate and then following some several steps we basically collapse and break apart
that tetrahedral intermediate and eventually we hydrolyze that peptide bond now one example of a
metalloproteins that is found inside our body is carboxy peptidase a and this is once again another example of a
digestive enzyme so we see that even though we have many different groups many different types of protease is we
have serine proteases cysteine protease --is a special protease --is metalloproteases and so forth all these
different types of proteases basically function in a very similar way what they ultimately do is they transform a bad
nucleophile into a good nucleophile and they transform a bad electrophile into a better electrophile and so that
ultimately we have a strong nucleophile attacking a very good electrophile and that's exactly what increases the rate
of that the rate of that reaction the rate of the hydrolysis of that peptide bond
Heads up!
This summary and transcript were automatically generated using AI with the Free YouTube Transcript Summary Tool by LunaNotes.
Generate a summary for freeRelated Summaries
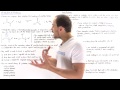
Understanding Proteases: The Powerful Enzymes in Protein Hydrolysis
Explore the mechanisms and functions of proteases in breaking down peptide bonds in our body.
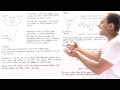
Understanding the Specificity of Serine Proteases: Kimotripsin, Trypsin, and Elastase
Explore the mechanisms behind serine proteases and their specificities in peptide bond cleavage.
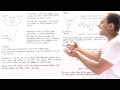
Understanding the Specificity of Serine Proteases: Kimot, Trypsin, and Elastase
Explore how serine proteases like Kimot, Trypsin, and Elastase cleave peptide bonds with specificity.
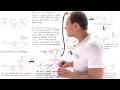
Understanding the Catalytic Triad in Chymotrypsin: Mechanism of Peptide Bond Cleavage
Explore how the catalytic triad of chymotrypsin promotes the cleavage of peptide bonds effectively.
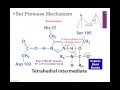
Understanding the Catalytic Mechanism of the Digestive Enzyme Chymotrypsin
Explore how chymotrypsin catalyzes the digestion of proteins through its unique mechanism.
Most Viewed Summaries
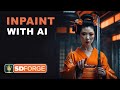
Mastering Inpainting with Stable Diffusion: Fix Mistakes and Enhance Your Images
Learn to fix mistakes and enhance images with Stable Diffusion's inpainting features effectively.
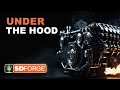
A Comprehensive Guide to Using Stable Diffusion Forge UI
Explore the Stable Diffusion Forge UI, customizable settings, models, and more to enhance your image generation experience.
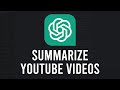
How to Use ChatGPT to Summarize YouTube Videos Efficiently
Learn how to summarize YouTube videos with ChatGPT in just a few simple steps.
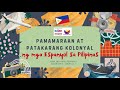
Pamaraan at Patakarang Kolonyal ng mga Espanyol sa Pilipinas
Tuklasin ang mga pamamaraan at patakarang kolonyal ng mga Espanyol sa Pilipinas at ang mga epekto nito sa mga Pilipino.
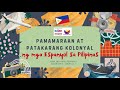
Pamamaraan at Patakarang Kolonyal ng mga Espanyol sa Pilipinas
Tuklasin ang mga pamamaraan at patakaran ng mga Espanyol sa Pilipinas, at ang epekto nito sa mga Pilipino.