Introduction
In the realm of thermodynamics, the Gibbs Free Energy formula plays a crucial role in understanding the spontaneity of reactions and processes. This article delves into the derivation of the Gibbs Free Energy formula, shedding light on the concepts of reversible and irreversible processes. We'll use a theoretical framework, involving entropy, enthalpy, and specific conditions that define these processes.
Understanding the Basics of Gibbs Free Energy
The Gibbs Free Energy (G) is defined as:
[ G = H - TS ]
where H is enthalpy, T is the temperature, and S is the entropy of the system. This equation helps identify whether a process is spontaneous or not. A negative change in Gibbs Free Energy (( \Delta G < 0 \)) indicates a spontaneous process.
Key Terms:
- Gibbs Free Energy (G): A thermodynamic potential that measures the usable energy in a system.
- Enthalpy (H): A measurement of heat content in a system at constant pressure.
- Entropy (S): A measure of the disorder or randomness in a system.
- Spontaneous Process: A process that occurs without external intervention or additional energy input.
Exploring Reversible and Irreversible Processes
To derive the Gibbs Free Energy formula, we need to distinguish between reversible and irreversible processes.
Reversible Processes
A reversible process is an ideal process that can be reversed without any net change in the system and the surroundings.
- Characteristics:
- Occurs infinitely slow.
- Always in equilibrium at each state.
- No energy losses due to friction or other irreversible factors.
As an example, imagine a piston-cylindrical system where a gas expands slowly. The system allows for energy transfer with a heat reservoir, keeping the process at a constant temperature (T).
Irreversible Processes
In contrast, an irreversible process cannot return to its original state without changes in the surroundings.
- Characteristics:
- Occurs rapidly and creates a gradient in the system.
- Leads to friction and energy loss, which contributes to heat generation.
For example, if we were to remove large blocks from the pistons instead of pebbles, the system would quickly transition to the new state without ever maintaining equilibrium.
Relation of Entropy in Both Processes
Both processes can lead to a change in entropy (ΔS).
- For the reversible process, the change in entropy is given by:
[ \Delta S_{rev} = \frac{Q_{rev}}{T} ]
where ( Q_{rev} ) is the heat absorbed by the reversible process.
- For the irreversible process, even though less heat is absorbed, the change in entropy is still expressed similarly:
[ \Delta S_{irrev} = \frac{Q_{irrev}}{T} ]
As established before, while both systems have the same change in entropy, the heat absorbed in the irreversible process (( Q_{irrev} )) is less than that in the reversible process (( Q_{rev} )), leading to an increase in entropy overall.
Total Change in Entropy of the Universe
For a reversible process, the total change in entropy of the universe (system + surroundings) is zero:
[ \Delta S_{universe, rev} = 0 ].
For irreversible processes, however, since there is an increase in entropy due to the irreversible nature and heat generation, we can show: [ \Delta S_{universe, irr} > 0 ].
Derivation of the Gibbs Free Energy Formula
Now, let’s see how we derive the Gibbs Free Energy equation from these discussions.
- Start with the relationship: [ Q_{irrev} - T * \Delta S_{irrev} < 0 ]
- Multiply through by -1: [ -Q_{irrev} + T * \Delta S_{irrev} > 0 ]
- Rearranging gives: [ Q_{irrev} < T * \Delta S_{irrev} ]
- By acknowledging that ( Q ) is equivalent to the enthalpy (under constant pressure):
- Thus, we receive: [ \Delta G = \Delta H - T * \Delta S < 0 ].
At this stage, we observe that a negative Gibbs Free Energy indicates a spontaneous reaction.
Conclusion
The exploration of Gibbs Free Energy through the lens of reversible and irreversible processes provides insight into the spontaneous nature of physical and chemical reactions. By dissecting these concepts, we discover the interconnectedness of entropy, heat transfer, and thermodyamical states that lead to the derivation of the Gibbs Free Energy formula. This rigorous approach not only clarifies how spontaneous reactions occur but also deepens our understanding of fundamental principles in thermodynamics.
I've hopefully given you a bit of a gut feeling behind where the formula of Gibbs Free Energy comes from. In this video I want to do something a little bit more
rigorous and actually, I guess you could say derive the formula. So to do that, let's just study two systems that have
the exact same change in entropy. To depict that, I'll get out our handy PV diagram. What we're going to do is we're going to compare two
systems. One that's this perfect, reversible system and one that's irreversible or a spontaneous system. So they're both going to start here.
They're going to go from this point on the PV diagram to this point right here. And before we go into exactly what they're doing, I think
it's a good review to kind of talk about what an irreversible process and what a reversible process really is.
An irreversible process is this theoretical thing where you have no friction, where you're always so close to equilibrium that you can always go backwards.
You're always-- you can kind of view that the reaction is never really proceeding forward or backwards. Although obviously it is moving, so it's a very-- it
actually doesn't really exist in nature, but it's a useful theoretical construct. So let me draw a little.
So if this is a-- I'll get the good old pistons out. So let's say this is an-- I'll do it twice. So that's my irreversible piston-- That'll be my
reversible, this is going to be my irreversible. Let me draw them. OK, and let me label them.
So this right here is going to be reversible. And this right here is going to be irreversible. Or it could also be considered spontaneous.
Using the key word. That's going to matter for Gibbs Free Energy. But all spontaneous reactions are irreversible.
So they're going to go from this state to this state. So in our reversible world, we have this little cap to our piston.
And we have our gas in here, exerting some pressure. And what we'd have is a bunch of pebbles. We have a bunch of pebbles out here.
And we slowly remove the pebbles one by one. And as we remove the pebbles, our piston-- or this movable ceiling up here-- will move upwards.
It'll move upwards. So the pressure will push up, but as we move up, we will have lower and lower pressure because the gases will bounce
into the surface less. And we'll have a higher, higher volume. So as we move each infinitesimally small grain of
sand, and we're going to do it super slow, that we're always infinitely close to equilibrium. We're going to move from this state to this state.
And even better, let's view that this is the first stage of our Carnot cycle. Let's say in either case, we're on top of a reservoir.
So in either case, both of these systems are on top of this infinite reservoir that has a temperature T1. And what that does it keeps the temperature constant.
So we're going to travel along an isotherm. Because normally if we were to remove these things and if we just allowed it to occur adiabatically, we would
actually lose temperature. We would actually lose average kinetic energy as work is done.
But in this case we have this reservoir. So heat is just going to be transferred to my system. Heat is going to be transferred.
So let me call that QR. Right? If the reservoir wasn't here, our temperature would go down.
But since we do have the reservoir, we will constantly be transferring heat. And we've seen this.
This is just the first phase of the Carnot cycle. We'll move along an isotherm like this. This is the reversible case.
And the only reason why we can even draw the state at every point here is because reversible processes are quasistatic.
They're always infinitely close to equilibrium. And when we say reversible, we're also saying there's no friction between this little piston in the cylinder.
That if we put a grain of sand back, it'll go exactly to where it was before. And no energy was lost because there was no
heat of friction there. So this is what the graph of the reversible process would look like.
Now what does the graph of the error irreversible-- Well, actually no. I won't draw the graph, but let's talk about what the
irreversible process is going to be like. So it's going to look similar. It's going to look like that.
It's going to have its gases there. But for the sake of an argument, to get from that state to that state, instead of moving the pebbles one by
one, let's say I have these big blocks. And when I remove one of these big blocks, I go from that state to that state.
But obviously all hell breaks loose. So I'm not really defined in this in between state. But I definitely go from that state to that state once I go
back to equilibrium. Now the other key thing in the irreversible process-- and every real process in our world is irreversible-- is
that you're going to have friction here. As this moves up, it's going to rub against the side of the container and generate heat of friction.
So let me call that heat of friction. So let me ask you a question. If, in this case, Q sub R had to be added to the system to
maintain its temperature, what's going to be the Q sub irreversible here? How much has to be added to this system in order to keep
it at the constant temperature T1? Will it have to be more or less than what was added to the reversible system?
Well this guy, as this piston moves up, he's generating some of his own heat. So if this was an adiabatic process, he wouldn't lose as
much temperature as this guy would. So he's going to need less heat from the reservoir in order to maintain his temperature.
At T1. In order to get to this point on the isotherm. Remember, this irreversible process, we don't know what
happens over here. He might be travelling on some crazy path. In fact, we can't even define the path, because it goes out
of equilibrium. So it's going to be some crazy thing. But we know it pops back on the PV diagram right there.
But because it's generating some of its own heat from the friction, it's going to need less heat from the reservoir. So let me write that down.
The heat absorbed by the reversible process is going to be greater than the heat absorbed by the irreversible process.
And that's because the irreversible process is generating friction. Fair enough.
Now what is the change in entropy for both systems? Well they both started here. And they both ended here.
And entropy is a state variable. So the change in entropy for the reversible process is going to be equal to the change in entropy for the
irreversible process. They're both going from there to there. And obviously the entropy has changed.
We're going from one state to another state. And the entropy, well I won't go too much into it. But let's ask another question.
What is the total change in entropy of the universe for the reversible process? So for the universe, that's going to be-- Our universe
here is the reservoir in our system. So let me write here. Reversible.
I don't want to run out of space. Let me see, I'll do it in a different color. So this is the reversible process.
So the change in entropy of the universe is equal to the change in entropy of our reversible process plus the change in entropy of-- oh, I already used R for reversible,
so let's call it the reserv-- well, the first three letters are the same, so let me call it of our environment. Right?
And then the reversible process, the change in entropy of our reversible process is the heat added for our reversible process.
And we can use this definition because it is a reversible process. It's that over T1.
And then what's the change in entropy of our environment? Well it's giving away Q sub R. Right?
So its heat absorbed is minus Q sub R. And, of course, it's at a constant temperature. It is a heat reservoir.
It's at T1. So it equals 0. It equals 0.
Interesting. So actually I should take a little side note here. That, the change in entropy of the universe for a reversible
process is 0. And actually that should make a lot of intuitive sense because the whole point of a reversal process is you could
go in this direction, or you could go in that direction. It's always so close people to equilibrium you can move in either direction.
And if the entropy was greater than 0 in one direction, it would have to be less than 0 on the other direction. So it wouldn't be able to go in the other direction by the
second law of thermodynamics. So it actually makes sense that the entropy of the universe-- the change in entropy of the universe, not
just of the system-- when a reversible process occurs, is 0. Let's see if we can relate that to the irreversible
process So if I wanted to figure out the change in entropy of the irreversible process-- What's the change in entropy of the irreversible process?
And then let me subtract from that the heat that was taken away from the reservoir, from the irreversible process, Q sub IR, and of course all this over T1.
What is this going to be equal to in relation to 0? Remember this is an irreversible, spontaneous process.
Well, this value, the irreversible process is starting here and going there. So its change in delta S is the exact same thing as that.
Change in delta S is the same as the reversible process. So these two things are equivalent. Now, I just told you that since there's some heat of
friction, this guy has to take in less heat from the reservoir than this guy. So if this-- and I wrote it here.
I mean, let me clean this thing up a little bit. I wrote it right here. Q sub IR, the heat that the irreversible process has to
take from the reservoir, because generating its own heat from friction, is less than the heat from the reversal process.
So this number, right here, is less than this number here. Or you could view it this way. This number here was equal to this.
So this number here is going to be less than this. So this has to be greater than 0 for the irreversible, spontaneous process.
Now let's just do a little bit of mathematics. So this is the heat that was essentially given to the irreversible system.
It's a minus here because this is the term. It's kind of taken away from the actual reservoir. So let's just do a little bit of-- let's just multiply all
sides of this equation by T1. And we get T1 times delta S of the irreversible process minus Q of the irreversible process is greater than 0.
Now, well, let's just-- So what is this? How can we say this? But let's actually just multiply both sides of this by
negative 1. And remember, this is true for any irreversible, spontaneous process.
If you multiply both sides of this by negative 1, you get this. The heat added to the irreversible process minus T
times delta S of the irreversible process is going to be less than 0. This is true for any
irreversible, spontaneous process. And at this point, this should look reasonably familiar to you.
When we wrote the Gibbs Free Energy formula, we said change in G is equal to delta H minus T times delta S. And we said that if this is less than 0, then we have a
spontaneous process. And this all makes sense. Because these two are equivalent to each other.
What's the only difference here? Here we wrote heat added to the system. Here we wrote change in enthalpy.
And I've done three or four videos right now, where I say that change in enthalpy is equal to the heat added to a system, as long as we're dealing with a constant
pressure system. So we get this result just by comparing a irreversible to a reversible system.
And this is true for all irreversible, spontaneous processes. And then if we assume that we're dealing with a constant
pressure system-- so you can forget a little bit of what I just drew, because we know that this is true, and we assume constant pressure-- then we get to this.
And then we know that if something is spontaneous, then this right here must be less than 0. So hopefully you found that a little bit interesting.
Actually I'll do one more point here. This kind of gels with the idea that second law of thermodynamics tells us that for any spontaneous process,
delta S is going to be greater than or equal-- or well for any spontaneous process, is going to be greater than 0. Because although this right here isn't the formal
definition of entropy, because we're not dealing with a reversible process here, you can kind of think of it that way.
And so, at least, on an intuitive level it gets you there, that we have delta S greater than 0. And I won't fixate on that too much, because what I did
earlier in the video is more rigorous than what I'm heading to right now. So hopefully that gives you a sense of where you can get to
the Gibbs Free Energy formula, and how it drives spontaneous reactions from just our basic understanding of what reversible and irreversible processes are, and how they
relate to entropy and heat exchange and enthalpy.
Heads up!
This summary and transcript were automatically generated using AI with the Free YouTube Transcript Summary Tool by LunaNotes.
Generate a summary for freeRelated Summaries
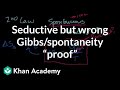
Understanding Gibbs Free Energy: The Correct Definition of Entropy
Explore the critical understanding of Gibbs free energy and the proper definition of entropy in thermodynamics.
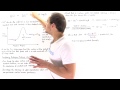
Understanding Gibbs Free Energy: The Key to Exergonic and Endergonic Reactions
Explore the significance of Gibbs Free Energy in determining spontaneous reactions, including exergonic and endergonic processes in biochemistry.
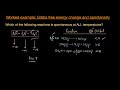
Understanding Gibbs Free Energy Changes in Chemical Reactions
Learn how to determine spontaneity in chemical reactions using Gibbs Free Energy and thermodynamic principles.
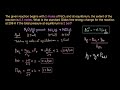
Understanding Gibbs Free Energy and Equilibrium Constants: An In-Depth Analysis
Explore the concept of Gibbs free energy and its relation to equilibrium constants through a detailed problem-solving approach.
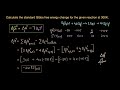
Calculating Standard Gibbs Free Energy Change for Chemical Reactions
Learn how to calculate the standard Gibbs free energy change for various chemical reactions step-by-step.
Most Viewed Summaries
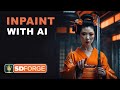
Mastering Inpainting with Stable Diffusion: Fix Mistakes and Enhance Your Images
Learn to fix mistakes and enhance images with Stable Diffusion's inpainting features effectively.
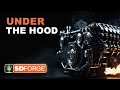
A Comprehensive Guide to Using Stable Diffusion Forge UI
Explore the Stable Diffusion Forge UI, customizable settings, models, and more to enhance your image generation experience.
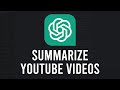
How to Use ChatGPT to Summarize YouTube Videos Efficiently
Learn how to summarize YouTube videos with ChatGPT in just a few simple steps.
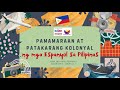
Pamaraan at Patakarang Kolonyal ng mga Espanyol sa Pilipinas
Tuklasin ang mga pamamaraan at patakarang kolonyal ng mga Espanyol sa Pilipinas at ang mga epekto nito sa mga Pilipino.
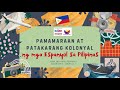
Pamamaraan at Patakarang Kolonyal ng mga Espanyol sa Pilipinas
Tuklasin ang mga pamamaraan at patakaran ng mga Espanyol sa Pilipinas, at ang epekto nito sa mga Pilipino.