Understanding the Role of Carbonic Anhydrase in Cellular Respiration and CO2 Transport
Heads up!
This summary and transcript were automatically generated using AI with the Free YouTube Transcript Summary Tool by LunaNotes.
Generate a summary for free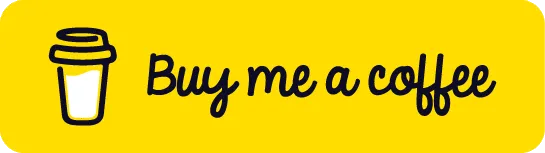
If you found this summary useful, consider buying us a coffee. It would help us a lot!
Introduction
In humans and other organisms, the process of cellular respiration plays a crucial role in transforming macromolecules into usable energy. This article delves deep into the biochemical processes involving carbonic anhydrase, an enzyme vital for maintaining CO2 levels within our cells. We will explore how this enzyme helps in metabolizing carbon dioxide (CO2) into bicarbonate ions, thus enabling efficient waste disposal and energy production.
Metabolism: The Breakdown of Macromolecules
Whenever we consume macromolecules such as proteins, carbohydrates, or lipids, our bodies initiate a breakdown process. These macromolecules are converted into their basic building blocks:
- Amino acids from proteins
- Glucose from carbohydrates
- Fatty acids from lipids
These smaller molecules are absorbed into the cells, where they undergo metabolism via aerobic cellular respiration. This process converts them into adenosine triphosphate (ATP), the energy currency of the cell, which powers various cellular processes.
The Challenge of CO2 Disposal
A crucial aspect of aerobic respiration is that it generates carbon dioxide as a waste product. However, as CO2 is nonpolar and not water-soluble, the body must effectively manage this waste.
- CO2 diffuses into the blood plasma, a polar environment, which makes it challenging to transport.
- Alveoli in the lungs facilitate the expulsion of CO2 from the body, but before this can happen, the CO2 must be converted into a stable form that can be dissolved in blood plasma; otherwise, it cannot be effectively transported.
Role of Carbonic Anhydrase in CO2 Transport
Once CO2 enters the red blood cells, it undergoes a critical transformation. This conversion, primarily facilitated by the enzyme carbonic anhydrase, transforms CO2 into the bicarbonate ion (HCO3-), which is soluble in blood plasma.
The Carbonic Anhydrase Enzyme
- Carbonic Anhydrase II is the most prominent form found in red blood cells.
- It accelerates the interconversion of carbon dioxide and water into bicarbonate and protons via hydration:
CO2 + H2O ⇌ H2CO3 ⇌ HCO3- + H+
This transformation is essential for maintaining acid-base balance in the blood and ensuring efficient CO2 disposal.
Mechanism of Carbonic Anhydrase
Active Site Structure
The active site of the carbonic anhydrase enzyme contains a zinc ion coordinated with various amino acid residues (notably histidines) and a water molecule. This active site is crucial for the enzyme's catalytic function.
- The zinc ion (+2 oxidation state) enhances the nucleophilicity of the water molecule, enabling it to better react with CO2.
Catalytic Action of Carbonic Anhydrase
- Water binds to the zinc ion at the enzyme's active site.
- The presence of zinc lowers the pKa of water, encouraging its dissociation into hydroxide (OH-) ions.
- The hydroxide ion acts as a powerful nucleophile and attacks the carbon atom of CO2, forming bicarbonate.
- This reaction mechanism allows for the rapid cycling of bicarbonate production, ensuring that CO2 levels in the blood remain stable.
Advantages of Metal Ion Catalysis
The mechanism of carbonic anhydrase serves as an example of metal ion catalysis. Here are the advantages:
- Increased reaction rates: The presence of the zinc ion enables faster conversion of CO2 to bicarbonate, meeting the metabolic demands of trillions of cells.
- Efficient waste management: The transformation into bicarbonate, a soluble form, ensures that CO2 can be carried in the plasma and safely expelled from the body.
Conclusion
In summary, carbonic anhydrase plays an indispensable role in cellular respiration by facilitating the conversion and transport of CO2 within the bloodstream. Through its catalysis via metal ion mechanisms, this enzyme not only enhances the rate of reaction for bicarbonate production but also contributes to the body's ability to maintain acid-base balance. Understanding this enzyme's function offers valuable insights into the broader processes of metabolism and energy production, emphasizing the intricate balance required for life.
anytime we ingest macromolecules for instance proteins carbohydrates or lipids we have to break these
macromolecules down into their individual form so we break them down into the amino acids the individual
sugar monomers glucose molecules and into fatty acids and ultimately these three different types of molecules amino
acids glucose molecules and fatty acids are brought into the cell and inside the cell the cell metabolizes breaks them
down into ATP molecules via process known as aerobic cellular respiration now these ATP molecules are used as
energy molecules to carry out different types of processes and reactions that take place inside the cells now the
problem with aerobic cellular respiration is it produces a waste by-product namely carbon dioxide and
carbon dioxide cannot actually be used by the cell in any useful manner and so all these trillions of cells which use
these food particles to metabolize them into ATP produce these co2 molecules and they essentially take these co2
molecules and dump them into the blood plasma why well because as the blood plasma circulates through our blood
vessels through our cardiovascular system eventually the co2 molecules will end up in the alveoli of the lungs and
it's the function it's the responsibility of these alveoli to basically expel all that co2 to the
outside environment out of our body and then the plants and trees can basically use the co2 to produce the sugar
molecules and then we can eat the sugar molecules and that process basically continues now the problem with this is
when all these trillions of metabolizing cells dump co2 into our blood plasma the co2 molecules themselves are nonpolar
but the blood plasm which consists predominantly of water molecules is polar and so the co2 in its co2 9
or form cannot actually dissolve inside the red blood plasma inside the blood plasma and so what happens is the co2
travels into the red blood cell now once inside the red blood cell we have the same problem again because the
cytoplasm of the red blood cell consists predominantly of water so just like the blood plasma the cytoplasm inside the
red blood cell is also polar and so the carbon dioxide cannot actually be stored inside the cytoplasm of the red blood
cells in its co2 form and so what ultimately has to happen is the cell has to carry out this particular reaction in
which we transform the nonpolar co2 molecule into its polar form bicarbonate ion and because this contains a full
negative charge it can easily dissolve inside the cytoplasm of the red blood cell and also inside the blood plasma of
our body so the problem with this reaction is so if we study the rate of this reaction in its on catalyzed form
at a pH of 7 and a button at body temperature would say 37 degrees Celsius we'll see that the rate of the reaction
isn't that great in fact the rate is nowhere close to what it should be to actually be able to meet the demands of
all the trillions of metabolizing cells found inside our body so to basically increase the rate enough value so that
it can keep up with the demands of our body what the red blood cells have is they have this enzyme we call a carbonic
anhydrase - so inside our body we have at least seven types of carbonic anhydrase enzymes but the one that is
found inside the red blood cells is known as carbonic anhydrase - now what we want to discuss in this lecture is
the active side of this carbonic anhydrase - and we want to discuss the mechanism that this molecule actually
uses to promote and cattle why's this reaction and what this reaction ultimately does is it hydrates
the carbon dioxide so water adds on to the carbon dioxide and that ultimately produced and that ultimately produces
the carbonic acid which dissociates into bicarbonate ion and the H+ ion so let's begin by taking a look at the active
side of carbonic anhydrase 2 so in our discussion on the mechanisms of enzymes we said that one mechanism that enzymes
use is known as metal ion catalysis and in metal ion catalysis the active side of the enzyme uses some type of metal
ion to basically form a strong nucleophile and that's exactly what we do in the active side of carbonic
anhydrase 2 so to see what we mean let's take a look at the following diagram so inside the active side of carbonic
anhydrase 2 and all of these carbonic anhydrase says we have a zinc metal atom and anytime any biological system uses a
zinc atom in nature the zinc atom always contains an oxidation state of positive 2 so it has a net charge of positive 2
and what that means is we have four different groups which are bound to that zinc atom so this is a zinc atom it has
a charge of positive two and it is bound to four different groups now three of these groups are these ring structures
that are part of the side chains of histidine residues so we have histidine residue 1 2 & 3 and they're bound to the
zinc as shown and the final group is a water molecule and this is the same water molecule that will react with the
carbon dioxide to ultimately produce the bicarbonate so the first question is what is the role that zinc actually
plays so what zinc actually does is by reacting with the water it transforms water into a much better nucleophile and
because water will be a much nucleophile as a result of this interaction it will be able to react
with the carbon dioxide and a much higher rate and so will produce these carb these bicarbonate ions as we'll see
in just a moment at a much higher rate so to see what we mean let's compare the pKa values of these two reactions so
remember the lower the pKa value the better the acid now if we take just plain water and we allow water to
dissociate into H+ ions and these hydroxyl ions the pKa value is fifteen point seven and this is a very high
value and what that were a relatively high value and what that means is this will not be a good enough acid and if
it's not a good enough acid it will not be very likely to produce this hydroxide ion and as we'll see in just a moment
it's the hydroxide ion that will be much more likely to act as a nucleophile and attack the carbon dioxide than the water
now what this binding does is because of this association this reaction the dissociation of that H+ ion to form the
hydroxide and the H+ greatly increases and so the pKa value decreases so we are because of this interaction between the
zinc atom and the water we basically decrease the PKA value of this reaction and by decreasing the PKA
value we essentially make it much more likely that the water will dissociate and produce that hydroxide and it's the
hydroxide that will be more likely to actually react with the carbon of the carbon dioxide so based on experimental
data we see that the binding of the zinc atom to water lowers the PKA value of water from fifteen point seven to seven
point zero and this makes water much more likely to give up its hydrogen ion and become a hydroxide ion
and why is that useful well it's useful because hydroxide ions are very very potent nucleophiles and so what that
means is once we form that hydroxide it will be much more likely to actually attack the carbon of that carbon dioxide
to ultimately form that bicarbonate on it and to see what we mean let's take a look at this reaction mechanism that
takes place inside the active site of carbonic anhydrase so in the first step we essentially form this complex that
consists of the water molecule bound onto that zinc so what happens is the oxygen of this water contains a partial
negative charge because remember it's more electronegative than either one of these H atoms and so because of that
partially negative charge on the oxygen it will be attracted to the positive two charge on the zinc and that will form
this bond as shown here now what that means is because this oxygen is interacting with the zinc the
interaction between the oxygen and either one of these H atoms will not be as strong and so the hydrogen one of
these hydrogen's will basically be much more likely to the sociate from that oxygen so water binds onto the metal
zinc atom the partially negative charge of oxygen is attracted to parch of to the positive charge of the zinc and this
decreases the ability of water to actually hold on to one of those H+ ions and this is precisely what facilitates
this process the dissociation of the H from this oxygen to basically form this molecule here and this is because the
pKa value is lowered from fifteen point seven to seven point O so this is very likely to actually take place now once
this takes place now there's room for the carbon dioxide to basically enter the active side the pocket of that
enzyme so this transforms a weak nucleophile into a very powerful nucleophile so once again as the H+ ion
basically leaves the oxygen we transform the water molecule up or nucleophile into a good nucleophile strong
nucleophile that hydroxide and now the carbon dioxide basically enters the active side now as the carbon dioxide
enters the active side what happens is the oxygen of that hydroxide attached to the zing acts as a nucleophile attacks
the carbon of that carbon dioxide and that displaces one of the PI bonds between carbon and oxygen and so now in
the next step we have a partial Network we have a full negative charge on one of the oxygens and that will be partially
stabilized by the zinc coming by the positive charge coming from that zinc and so we have this interaction shown in
orange that is stabilizing this structure here so in this step we see the highly nucleophilic oxygen attacks
the carbon of the carbon dioxide forming this bicarbonate intermediate shown here and once we form this intermediate
because of the instability of this intermediate and because we'll see another water molecule moving into the
active side that water molecule will essentially displace this entire molecule and that water molecule will
attach onto the zinc displacing and replacing this bicarbonate and that will kick off that bicarbonate and will and
will go right back to this stage here and so this reaction basically cycles back and forth and it takes place very
very quickly and so every time this takes place we produce a bicarbonate so we transform our carbon dioxide into the
bicarbonate which then dissolves into the cytoplasm inside red blood cells and into the blood plasma inside our
cardiovascular system so we see that the protease as we discussed previously we're basically examples of covalent
they used the metal ion catalysis but in the case of carbonic anhydrase these are examples of molecules which always use a
metal atom namely the zinc atom and so these carbonic anhydrase --is basically use the mechanism we know as metal ion
catalysis they use the metal ion to basically create a much better nucleophile out of the water and that
allows the hydroxide the better nucleophile attendent attack the carbon of that carbon dioxide to ultimately