Introduction
In the fascinating world of biochemistry, enzymes play a crucial role in catalyzing various biochemical reactions. One such enzyme is Kimot Tron, a sering protease renowned for its ability to cleave peptide bonds specifically at the carboxy end of large hydrophobic non-polar amino acids. This article delves into the mechanics of Kimot Tron, including its catalytic Triad, specificity mechanisms, and comparisons with other proteases in the body.
The Catalytic Triad of Kimot Tron
At the heart of Kimot Tron’s function is the catalytic Triad, which comprises three essential amino acids: aspartate, histidine, and cysteine. This collection of residues collaborates effectively to promote the cleavage of peptide bonds, enabling the enzyme's catalytic power.
Role of the Catalytic Triad
- Aspartate: Participates in the nucleophilic attack on the peptide bond.
- Histidine: Acts as a general base, facilitating the deprotonation of the cysteine residue.
- Cysteine: Functions as the nucleophile, attacking the carbonyl carbon of the peptide bond.
The interaction of these three residues is fundamental to the function of Kimot Tron, allowing it to cleave specific peptide bonds effectively.
Understanding Specificity
Despite possessing the same catalytic Triad as other serine proteases, Kimot Tron’s specificity depends largely on the structural characteristics of its active site, particularly the S1 pocket. The shape and composition of this pocket determine which substrates Kimot Tron can efficiently interact with and cleave.
The S1 Pocket
The S1 pocket of Kimot Tron is designed to accommodate large, hydrophobic, and non-polar amino acids. Key features include:
- Long and Deep Structure: The shape allows for long amino acid side chains to fit snugly into the pocket.
- Hydrophobic Environment: As the S1 pocket is predominantly hydrophobic, it minimizes electrical repulsion, favoring the binding of non-polar amino acids.
This design specifically enables binding and cleavage of residues such as methionine, phenylalanine, alanine, tyrosine, and tryptophan.
Variability Among Serine Proteases
The family of serine proteases includes several members, each exhibiting unique substrate specificities despite sharing the catalytic Triad. Aside from Kimot Tron, key examples are trypsin and elastase.
Key Differences in Active Sites
The differences among these proteases arise from slight variations in the structure of their respective S1 pockets, impacting their specificity:
- Trypsin:
- Cleavage at the carboxy end of lysine and arginine.
- Contains a negatively charged aspartate that stabilizes positively charged side chains, allowing it to recognize and cleave bonds after basic amino acids.
- Elastase:
- Cleavage at the carboxy end of small, hydrophobic amino acids such as glycine, alanine, and valine.
- The presence of valine residues at the bottom of the S1 pocket restricts its accommodation capabilities to only small and hydrophobic side chains.
Mechanism of Cleavage
All three proteases - Kimot Tron, trypsin, and elastase - utilize the same fundamental mechanisms of co-covalent catalysis and acid-base catalysis. Understanding these catalytic mechanisms is essential for grasping how different enzymes achieve their respective functions despite having similar catalytic Triads.
Summary of Specificities
- Kimot Tron: Cleaves at the carboxy end of large hydrophobic amino acids.
- Trypsin: Cleaves at the carboxy end of positively charged amino acids (lysine, arginine).
- Elastase: Cleaves at the carboxy end of small hydrophobic amino acids.
Conclusion
In summary, Kimot Tron exemplifies the complexity and specificity of serine proteases in biochemistry. Its ability to cleave peptide bonds is intricately tied to the composition of its catalytic Triad and the unique structural features of its S1 pocket. By understanding these compelling aspects, we can gain greater insight into the roles enzymes play in biological processes, paving the way for future research into enzymatic functions in health and disease.
kimot Tron is a sering protease and it has the ability to catalyze the cleavage of peptide bonds on the carboxy end of
large hydrophobic non-polar amino acids for instance kimot Trion Cleaves at the carboxy end of methionine phenol alanine
tyrosine as well as tryptophan now as we discussed previously it's the presence of the catalytic Triad inside the active
side of kyrion that actually gives it the power of catalysis gives it the ability to actually cleave those peptide
bonds so remember the catalytic Triad is basically this collection of three individual residues aspartate histadine
and cine which work together to basically promote the cleavage of those peptide bonds now the question Still
Remains what exactly gives kyrion its specificity what gives Kim trips in the ability to only cleave on the carboxy
end of specific amino acids those amino acids that contain bulky hydrophobic side chain groups now to answer this
question we actually have to study the shape of the active side the structure of that enzyme if we examine the active
side of that enzyme we're going to find something called the S1 pocket and the S1 pocket in kimat tripid is basically
that real region to which that side chain group will actually move into and if we examine the shape and structure of
the S1 pocket we're going to find that it's relatively long so relatively deep and mostly hydrophobic so non-polar and
because of that structure of the S1 pocket only those amino acids that contain side chain groups that are long
nonpolar do not have any charges will actually be able to fit into that pocket into the active side without creating
too much electric repulsion so amino acids such as methine phenol alanine tyrosine and tryptophan so once again
it's the catalytic Triad in the active side it's the presence of these three individual amino acids that give kimot
Tron its catalytic power the ability to actually catalyze the cleavage of those peptide bonds but it's this long and
narrow shape and the fact that it's mostly hydrophobic it's the shape of the S1 pocket that actually gives katriin
its specific nature its specificity to cleave only on the carboxy and of specific side chain
groups now as we discussed in our uh study of proteases we basically said there are many different types of
proteases that exist in inside our body and inside nature now so far we focused on seing proteases and we use kimot
tripson as the prototypical sering proteas but of course in our body in our digestive system for example we have
many other examples of searing proteases the question is what is the mechanism that these other seing proteases
actually Ed to cleave peptide bonds well it turns out other serum proteases also use this same catalytic Triad and what
that means is they also carry out the catalysis process by using the same exact mechanism namely Cove valent
catalysis and acidbase catalysis and two examples of other serum proteases inside our digestive system that also contain
the same catalytic Triad is Tron as well as elastase so kimat Trion is is not the
only sering protease that utilizes this catalytic Triad in fact Tron and elastase are two other sering proteases
found in our digestive system that use this same exact catalytic Triad and therefore the same exact mechanism of
catalysis that we spoke about in the previous lecture now the question is why do we have different types of CM
proteases inside our body body and the question is what exactly differentiates tripson elastase and kimat Trion if they
have the same exact catalytic Triad well remember it's the catalytic Triad that gives the enzyme its catalytic power
that gives the protease the ability to cleave those peptide bonds but it's the shape of that active side the S1 pocket
that determines the specificity of that protease the type of eptide Bond it actually breaks and so the difference
between Kima trips and tripson and El lastas is not in the type of catalytic Triad used but it's in the shape of that
particular S1 pocket the structure of that S1 pocket as we'll see in just a moment as a result of a slight variation
in the S1 pocket of tripson and Al last days we see that these other serm proteases cleave other amino acid so all
the trips in El last days use the same mechanism so coent catalysis and acid-based catalysis which we spoke
about in the previous lecture they differ in their specificity and that has to do uh and that has to do to the fact
that there is a slight structural difference in the S1 pocket in tripson as well as alasas and let's see what
these differences are and what they result so let's begin with Trion so Tron catalyzes the cleavage of peptide bonds
on the carboxy end of Lysine and Arginine and if you recall lysine and Arginine both contain positive charges
on their side chain groups now the question is why is this true so tripson uses the same exact catalytic Triad that
kimat Trion uses but what gives this Tron the difference in specificity well if we examine the S1
pocket of tripson at the bottom of that S1 pocket we're going to see we're going to see a residue that we don't see in
the S1 pocket of Kima tripson at the bottom of the tripson S1 pocket we have a negatively chared side chain that came
from the aspartate that we see in tripson and we don't see in Kima tripson so as a result of the negatively chared
side chain of aspartate 189 that is found at the bottom of the S1 tripson pocket we see that this tripon only
Cleaves at the carboxy end of those amino acids which are long and contain a positive charge at the end and these
happen to be lysine and Arginine so if we examine the following hypothetical polypeptide we have glycine this one
here we have lysine this one here we have glycine this one we have Arginine this one and we have glycine this one
now the only ones that contain positive charges are these two amino acids and only these amino acids will be able to
actually fit into the pocket of Tron and at the end will be able to actually interact in a stabilizing manner so the
positive charges of these two side chain groups will interact with the negative charges of of the of this aspartate 189
and that and that will create a stabilizing effect it will neutralize the net charge in that space and that
will create a very stable effect so at the bottom of the active side of tripson is an aspartate residue and the negative
charge of the aspartate side chain group will stabilize those amino acids that contain side chain groups with positive
charges namely the lysine and the Arginine and so the only bonds that tripson will be able to cleave are this
Bond and this Bond here so at the carboxy end of Lysine and Arginine so we see that a very tiny
variation in the structure of the S1 pocket in tripson gives tripson uh a different specificity than that of kimat
Trion and finally let's move on to AAS days so if we examine the S1 pocket of elast days we'll see the presence of two
additional valine and valine if you recall are these small or valine molecules have these small relatively
small hydrophobic chains and notice where these two valins are positioned they're positioned opposite of each
other and they essentially play the role of blocking the majority of the bottom portion of that S1 pocket and so what
that means is when the side chain group of the amino acid moves into the S1 pocket it cannot actually occupy this
space here because this blocks as a result of the stair Kinder of the hydrophobic properties of these side
chain groups and so only those amino acids that have relatively small side chain groups and which are non-polar so
uncharged will be able to fit into this pocket and so we see that elastase Cleaves peptide bonds on the carboxy end
of small hydrophobic amino acids such as glycine valine alanine valine alanine Lucine and isoline as well as Seine so
if we have this hypothetical polypeptide chain we have glycine lysine alanine phenol alanine glycine so the only ones
which have a small hydrophobic side chain are glycine alanine as well as glycine at the end so
the only two peptide bonds that are going to be cleaved are this peptide bond so on the carboxy end of glycine as
well as this one on the carboxy end of alanine so lysine and phenol alanine are not small ones this one has a large long
one and it's positively charged although this one is is uh non-polar hydrophobic it's too large to actually fit into this
pocket because these two valins block the majority of that pocket and even though this is a glycine there's no bond
on the car boxal side and so nothing will be cleaved on this side by last days and so we see that two Valiant
residues found in the S1 pocket of el lastes block off the majority of the pocket in El lastes and this allows the
last days to only cleave small hydrophobic residues and so we see that the majority of the serum proteases
found inside our body for instance kimat Trion Tron as well as elastase although they use the same catalytic Triad to
basically catalyze the cleavage of the peptide bonds they actually differ in the types of amino acids that they
cleave types of peptide bonds that they cleave as a result of these structural differences and shapes in the S1 pockets
of their active sides
Heads up!
This summary and transcript were automatically generated using AI with the Free YouTube Transcript Summary Tool by LunaNotes.
Generate a summary for freeRelated Summaries
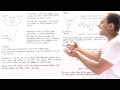
Understanding Kimotripsin: The Key Serine Protease and Its Specificity
Explore the functionalities and specificity of Kimotripsin, a significant serine protease responsible for peptide bond cleavage.
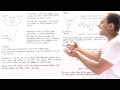
Understanding Kimotripsin: The Sering Protease's Catalytic Mechanism and Specificity
Explore Kimotripsin's unique catalytic mechanism and specificity for peptide bonds. Discover the role of the S1 pocket and catalytic Triad.
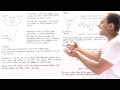
Understanding the Specificity of Serine Proteases: Kimot, Trypsin, and Elastase
Explore how serine proteases like Kimot, Trypsin, and Elastase cleave peptide bonds with specificity.
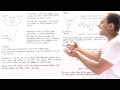
Understanding Kimot Tripsin: The Searing Protease and Its Specificity
Discover the unique properties of Kimot Tripsin and its catalytic mechanism in peptide bond cleavage.
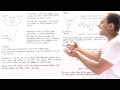
Understanding Kimot Tripsin: Mechanisms and Specificity of Serine Proteases
Explore kimot tripsin’s role in peptide bond cleavage and the specificity of serine proteases in biological systems.
Most Viewed Summaries
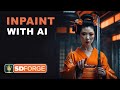
Mastering Inpainting with Stable Diffusion: Fix Mistakes and Enhance Your Images
Learn to fix mistakes and enhance images with Stable Diffusion's inpainting features effectively.
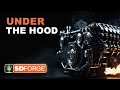
A Comprehensive Guide to Using Stable Diffusion Forge UI
Explore the Stable Diffusion Forge UI, customizable settings, models, and more to enhance your image generation experience.
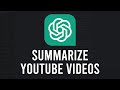
How to Use ChatGPT to Summarize YouTube Videos Efficiently
Learn how to summarize YouTube videos with ChatGPT in just a few simple steps.
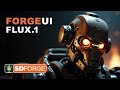
Ultimate Guide to Installing Forge UI and Flowing with Flux Models
Learn how to install Forge UI and explore various Flux models efficiently in this detailed guide.
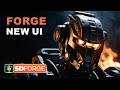
How to Install and Configure Forge: A New Stable Diffusion Web UI
Learn to install and configure the new Forge web UI for Stable Diffusion, with tips on models and settings.